Decarbonize transport with battery electric vehicles
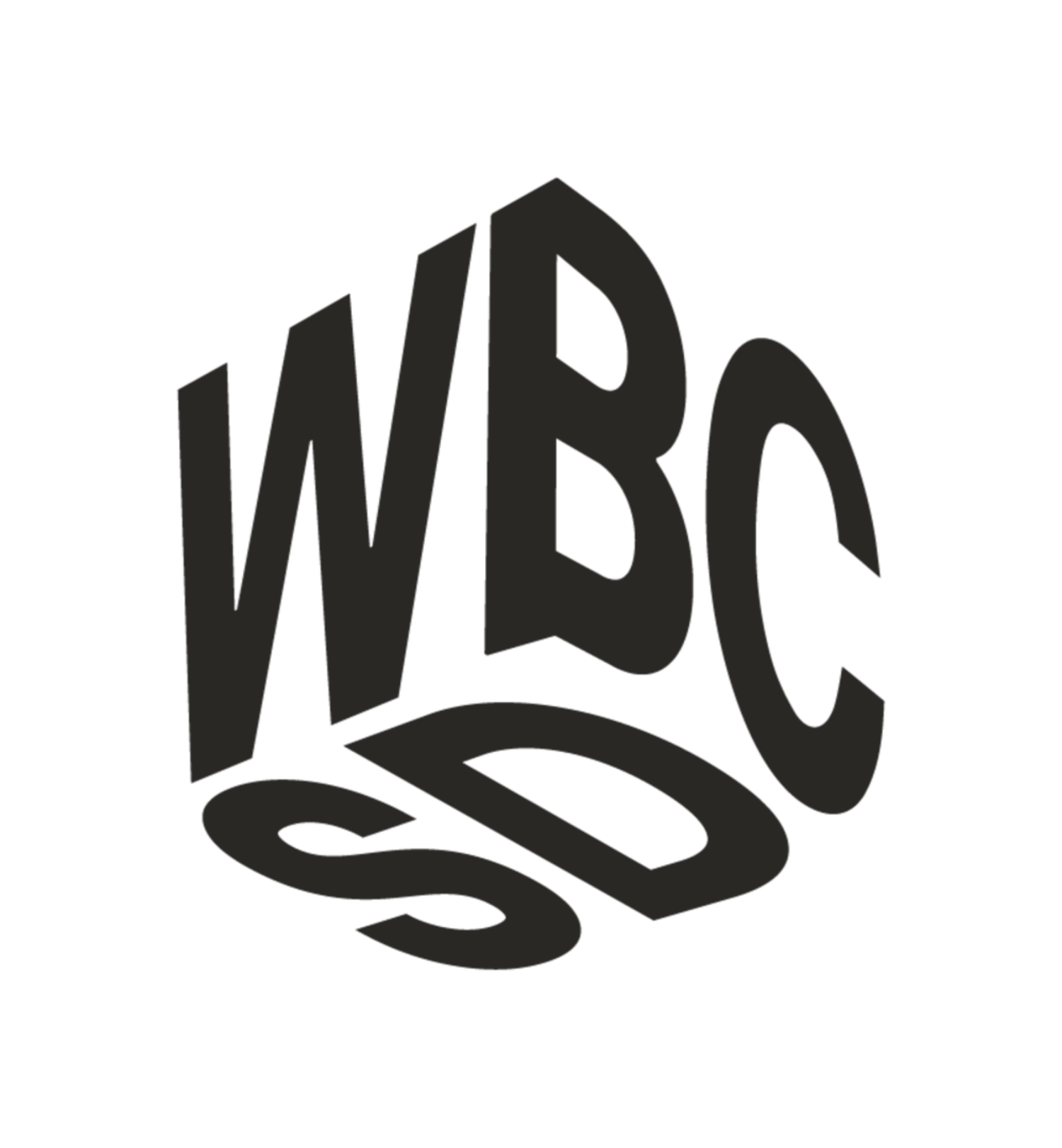
Summary
Switching to battery electric vehicles (BEVs) is an alternative to internal combustion engines (ICEs) which can reduce direct fuel combustion emissions from fossil fuels
Key resources
Solution
The transport industry accounts for 16.2% (1) of annual global carbon dioxide emissions, producing approximately 7.3 giga tonnes of CO2 emissions per year (2) with passenger vehicles representing around 40% of these emissions (3.0 GtCO2e/year). However, the predominant powertrain system for passenger vehicles is still based on the internal combustion of fossil fuels.
The increased use of battery electric vehicles (BEVs) as an alternative to internal combustion engines (ICEs) can reduce direct fuel combustion emissions from fossil fuels.
Total life cycle emission reduction potential is expected to reach a maximum of 65% of conventional ICE counterparts (3). While further BEV adoption may also increase electricity-related emissions in the short term, use phase-related emissions of BEVs are expected to decrease in the mid-to-long-term, for example, through increasing the share of renewable energy sources in BEV production.

Usage
Market demand for BEVs has significantly increased in recent years (+231% Q/Q, Q2 2021 within EU). Demand has been driven primarily by more advanced electric vehicles becoming available, more policy incentives for the electrification of the automotive industry, and greater availability of charging stations. Customers are also increasingly considering electric vehicles as achievable and an attractive substitute to internal combustion engine offerings.
Forecasts expect BEVs to account for 75 to 95% of new passenger vehicle sales by 2030 globally. Under current trends, the EU would adapt and enable electrified automotive infrastructure more rapidly over the next decade than other regions, which would follow by around 2035.
Image: EV sales

Impact
Climate impact
Targeted emissions sources
Switching from ICE to BEV targets emissions along all life cycle phases:
Manufacturing phase
Use phase
End-of-life treatment phase
This typically impacts organizations’ Scope 1 and Scope 2 emissions, e.g. through increased electricity usage, while also impacting Scope 3:
Category 1 (purchased goods and services)
Category 11 (use of sold products)
Category 12 (end-of-life treatment of sold products)
Decarbonization impact
Manufacturing electric vehicles leads to higher initial carbon dioxide emissions by 75% (9.7 tCO2e for BEV and 5.5 tCO2e for ICE) due to increased emission intensity of materials used for battery production and overall higher vehicle weight caused by battery/powertrain components. Sustainable sourcing and usage of green, recycled materials can help manufacturers to achieve a lower production carbon footprint.
Use phase carbon dioxide emissions of electric passenger vehicles are dependent on the electricity mix in the country of use. While in some regions, they enable CO2 emissions reduction of roughly 90% (e.g. Sweden), in other countries that rely more heavily on coal-based electricity production, their use can lead to an overall increase of use phase emissions. To enable the full environmental potential of electric vehicles, fueling EVs with clean, renewable power is a necessity.
End-of-life treatment CO2 emissions for electric passenger vehicles can be substantially higher, as component recyclability increases due to higher end-of-life value for used materials.
Business impact
Benefits
Lower life cycle emissions, lower operating costs, exemption from clean air zones charges/fees, tax breaks and regional subsidies, the possibility of free parking in cities, increased resale value.
Costs
Impact on operating costs
BEVs have the lowest operating cost of all possible powertrain solutions for passenger vehicles – lower by 30 to 55% percent (in $US/km).
Investment required
The capital investment for passenger BEVs is roughly 28% higher than for ICEs. As the technological development of electric vehicles advances and cost competitiveness rises, the EV investment cost is expected to fall by around 15-20% by 2030, hence to almost reach up-front cost parity.
Eventual subsidies used
Regional and country-specific subsidies apply based on location of use.
Indicative abatement cost
Abatement cost for passenger transportation (compared to ICE vehicles):
100 to 200 US$/tCO2e in 2022
-300 to -100 US$/tCO2e by 2030
Impact beyond climate and business
Co-benefits
Lower noise level, integration of renewables within the charging infrastructure, lower emission levels and health benefits in densely populated regions, circular economy implementation in end-of-life treatment, reduced noise pollution, second-hand electric market opportunities.
Potential side-effects
Possibility of general increased carbon dioxide emissions due to the country’s available electricity mix, leach of hazardous raw materials in local communities during extraction for electric vehicles, higher load on electrical networks.
Implementation
Typical business profile
All institutional, private businesses or individuals interested in switching to electric vehicles in all geographies.
Approach
The adoption of electric passenger vehicles should be recognized at a local level, based on available charging infrastructure, associated costs, subsidies, tax breaks, and maintenance center accessibility.
Consider vehicle specification (e.g. range, power, price) and the possibility of installing a charging station at home/work or local charging infrastructure distances.
Stakeholders involved
Company functions: All functions
Main providers: Largest electric vehicle manufacturers by market cap: Tesla, BYD, SAIC, VW Group, Hyundai Motor Group.
Key parameters to consider
Solution maturity: well-known, established solution across all global markets
Lifetime: around 10-15 years
Technical constraints or pre-requisites: lower range vs ICE, higher initial cost of investment, vehicle specification
Additional specificities (e.g., geographical, sector or regulation): charging infrastructure irregularity, country-specific electricity mix
Eventual subsidies available: dependent on the country of use
Implementation and operations tips
Implementation of electric vehicles across all geographies is progressing. Over the next decade, multiple challenges must be solved, for example charging infrastructure capacity, availability, speed, vehicle range constraints, and cost competitiveness vs ICEs.
When considering an investment in an electric vehicle, be mindful of the availability and speed of local chargers, your travel patterns, and the range required for daily usage.
To lengthen the lifetime and maximize battery capacity avoid leaving the vehicle on low battery levels for long periods of time. Extreme temperatures can degrade battery capacity. Planning for charging ahead of time for longer trips is fundamental.
Going further
Main sources
Further readings